|
Hi friend,
Welcome to the Watch This Spacetime newsletter! There are three possible reasons you’re receiving this:
-
You signed up for my newsletter literally years ago when I had previous ambitions to send updates and was thwarted by life things.
-
You signed up for this new newsletter, specifically!
-
Some terrible error has occurred, for which I am deeply sorry.
This new version of the newsletter exists to collect some of the physics/cosmology stuff I’ve produced in a regular dispatch, and to share some casual, not-otherwise published thoughts. You can sign up or cancel any time, and it’s all free (though there are ways to offer support if you really want to – see bottom of message for details!). And feel free to write back and share your thoughts/reactions/questions – you can treat it kind of like Twitter, but not hijacked by an egomaniacal billionaire with problematic motivations. |
-Katie |
|
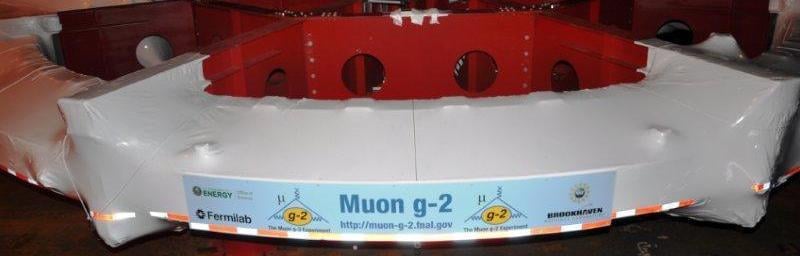 |
|
|
Speaking of physics hot takes, have you seen the news about the latest results from the Muon g-2 experiment at Fermilab?
(This bit is a giant block of text; scroll down for links and upcoming events.)
It first crossed my newsfeed in the form of a BBC News article announcing, “Scientists at Fermilab close in on fifth force of nature.” Quite the claim! And quite the contrast to the second article I saw, in Nature Magazine, which stated, “Dreams of new physics fade with latest muon magnetism result.” It’s pretty rare to see such a massive spread in interpretations of a physics result. So what gives? It all comes down to the interplay between theory and experiment in particle physics, and how uncertainty in each is treated.
The experiment is a relatively straightforward one. The Muon g-2 experiment is a giant ring that sends muons – tiny particles that behave like more massive, less stable versions of electrons – around in circles to measure their magnetic properties. (Technically this experiment uses antimuons, but the news articles rarely mention that because to physicists that just means there’s a minus sign somewhere but otherwise the results are the same.)
The “g-2” name comes from the muon’s “g-factor,” related to its magnetism, and the fact that in the most straightforward theoretical calculation one can do, the number should be equal to 2 (for which g-2 would equal zero). But the g-factor is definitely NOT equal to 2, for reasons that have to do with a weirdness of quantum physics: it turns out muons interact with a whole sea of ghostly “virtual particles” all the time, each of which tweaks its g-factor just a bit.
In principle, the Standard Model of Particle Physics tells us what those virtual particles should be, and how they should be poking at the muon. We should be able to calculate the difference between the g-factor and 2 just by knowing how the Standard Model works. The reason this is an important thing to calculate and measure is that if that difference, g-2, is off from what we expect, it could mean that the sea of virtual particles contains something new – something not already included in the Standard Model. And indeed, Fermilab’s g-2 experiment sees a discrepancy between their predictions and the experimental results, with the latest data pushing that discrepancy past the gold-standard “5-sigma” threshold indicating that there’s less than a one in a million chance that the result is a statistical fluke. New particles can point to the possibility of new fundamental forces of nature, hence the BBC headline: taken at face value, this seems to be an open-and-shut case of the discovery of physics beyond the Standard Model!
So why the pessimism from other reports?
The problem is not that the experiment is too uncertain. The problem is the theory. As it happens, calculating the anomalous magnetic moment of the muon (the thing that makes the g-factor different from 2) is extremely complicated. It’s so complicated, in fact, that physicists can’t get a straightforward answer from the theory just using pen-and-paper or even traditional numerical calculations. They have to either incorporate other experimental results to get hints about some of the more difficult terms, or throw the whole problem into a supercomputer calculation that pixelizes space and time and physical interactions and tries to use hardcore brute-force numerical methods to make sense of it all. The former approach is called the “R-ratio method,” and the latter is lattice QCD. (QCD = quantum chromodynamics, the theory of the strong nuclear force.)
In 2021, when hints of Fermilab’s g-2 discrepancy were first announced, the result was compared to an R-ratio calculation that put together the best computational methods and experimentally derived add-ons available at the time. That’s the Standard Model result that the new results are discrepant from at the 5-sigma level. But even back then, freshly performed lattice QCD calculations were throwing everything into confusion by coming up with an expected number for g-2 that was different from the previous estimate and much closer to what the experiments were finding. If those calculations were the right Standard Model answer, there would be no need for new physics at all. There was still a big mystery as to why the R-ratio calculation disagreed with lattice QCD (which itself could be a major hint of something cool going on!), but if one trusted lattice QCD, Fermilab’s experimental result became much less exciting.
Since then, things have gotten even more murky: a new measurement of one of the factors in the R-ratio calculation seems to be able to push even that one into a range that’s in much closer agreement to the Fermilab experimental result.
The upshot of all this is that now we don’t know whether to interpret the experimental result as a first glimpse of exciting new physics or just one more point on the board for the good old Standard Model.
As confusing as it might be to a non-physicist trying to parse all these apparently contradictory headlines, it’s a good reminder that there are two sometimes equally difficult parts involved in testing a theory: doing the experiment, and calculating exactly what the theory says the experiment ought to see. When it comes to QCD, the theory is so complicated that the precision of the experiments is currently outpacing that of the theoretical expectation. Whether this means we’ll be able to continue to use experiments to better understand the theory and develop new computational tools, or whether what we find will ultimately point us in the direction of a new theory entirely, is yet to be seen. Stay tuned!
Further reading:
|
|
|
|
|
|
 |
Recent articles, interviews, and features. |
|
|
Quantum 101 Video Series |
Welcome to the quantum realm! Join me in these 10 short forays into the weird, wonderful world of quantum science. |
Watch Here |
|
|
|
Joy of Why Podcast: How Will The Universe End? |
Our universe has a beginning. And someday, it will have an end too — but which one? |
Listen Here |
|
|
|
BBC Science Focus |
Why we don’t have to worry about being sucked into the supermassive black hole at the centre of the Milky Way |
Learn More |
|
|
|
Great Mysteries of Physics Podcast: Is There A Multiverse? |
The multiverse idea has long been an inspiration for science fiction writers. But does it have any basis in science? And if so, is it a concept we could ever test experimentally? Featuring perspectives from me and other physicists. |
Listen Here |
|
|
|
 |
Mark your calendar for upcoming talks and events. |
Starfest 2023
"A Map of Cosmic Time"
August 19th, 2023
Ayton, Ontario, Canada
In Einstein’s universe, space and time merge and morph. In this presentation, we’ll explore how gathering data and about the distant universe can allow us to directly observe a timeline of our cosmic past, and how new theories and observations are giving us the tools to extend that timeline out into the future.
Secure your spot. |
|
U Toronto Astro Tours
"Physics at the End of the Universe"
August 24th, 2023
University of Toronto
The Big Bang theory tells the story of the beginning of the Universe, our cosmic home for the last 13.8 billion years. But how does the story end?
Find out more. |
|
|
|
|
 |
Previous articles, interviews, and other content you may have missed. |
|
|
Constructs: A commissioned science poem with voiceover and images, to showcase animations from Finger Industries
|
Watch Here |
|
|
|
BBC Science Focus: Why Schrödinger's Cat is still the most controversial thought experiment in science
|
Read Here |
|
|
|
 |
sometimes random physics things get stuck in my head |
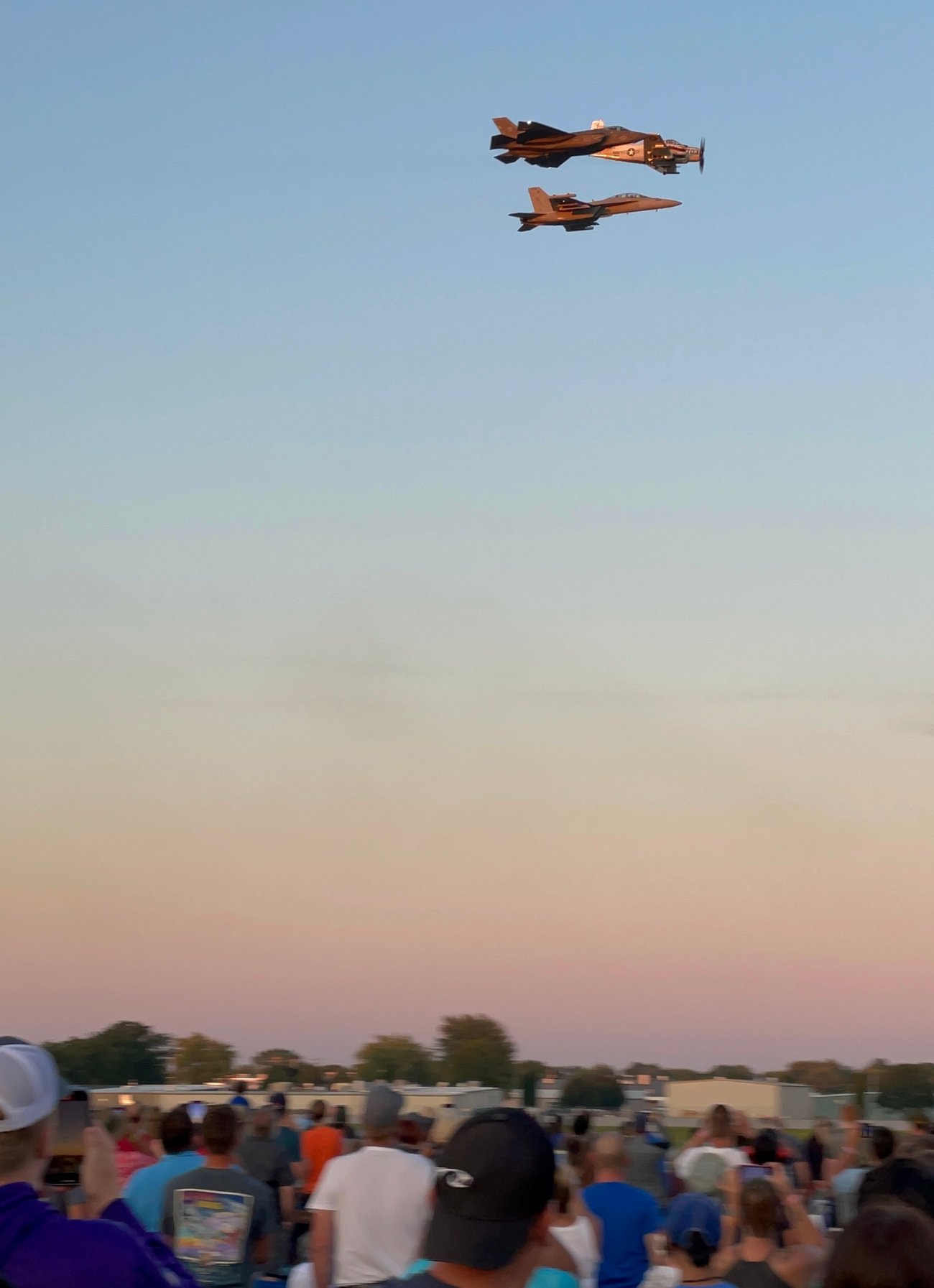 |
|
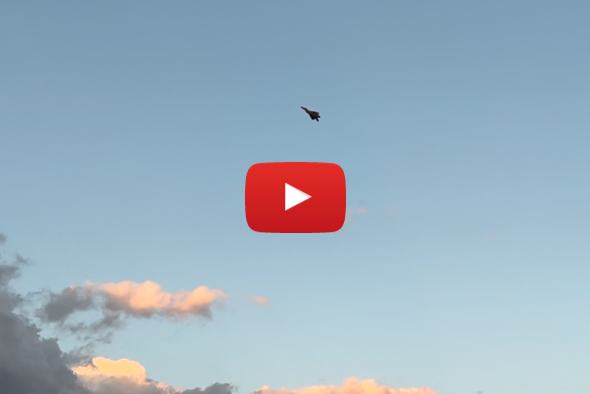 |
Angle of Attack
I've been to a couple of air shows and one of the things that captivates me the most is when a fighter jet demonstrates a "high-alpha pass": a level flight maneuver at the slowest possible airspeed. It's a great demonstration of the way in which lift, drag, and thrust all work on the plane's surfaces, and it just looks so weird. The basic idea is that for a plane with a lot of thrust, they have to have a really high angle of attack (angle between the wing and the air) in order to go slow -- it massively increases drag, and as long as the angle isn't too high and there's still enough thrust, the lift can be enough to keep the plane flying. It's especially stark when the modern fighter jets fly in formation with old warbirds, because the warbirds are completely level, absolutely booking it, and the jets are leaning back on their tails trying desperately not to fall out of the sky. |
Learn More: Angle of Attack |
|
|
|
|
|